Unveiling the Fascinating Dynamics of Membrane Channels in Cellular Physiology
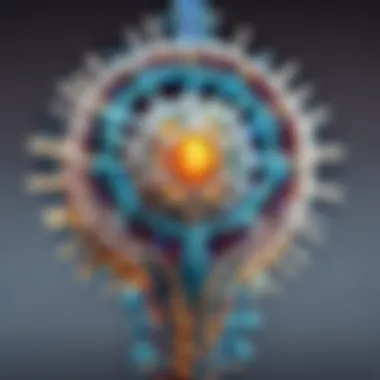
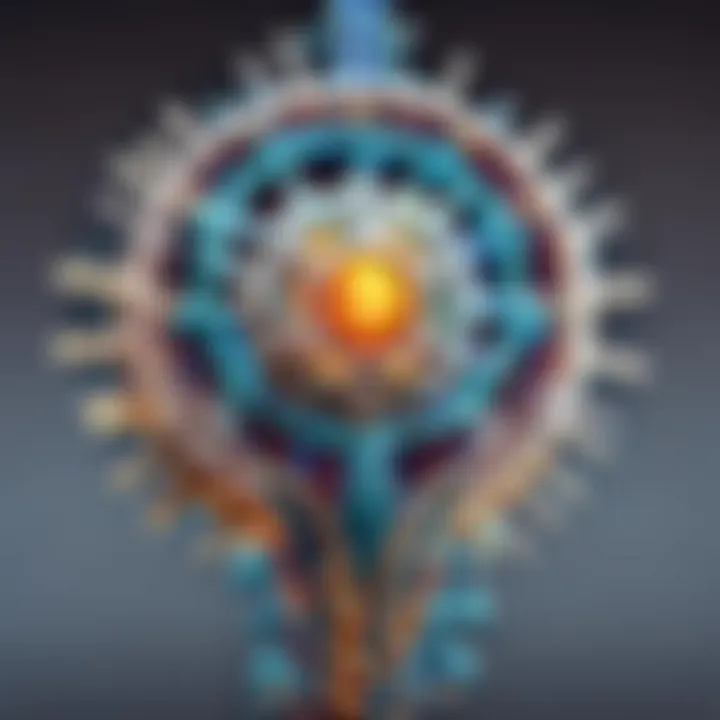
Exploring the Depths of Membrane Channels in Cellular Mechanics
The realm of membrane channels harbors a complexity that underpins crucial aspects of cellular physiology, exerting a profound influence on the orchestration of cellular functions. This article embarks on a meticulous journey through the intricate landscape of membrane channels, unraveling their structural nuances, functional significance, and intricate interplay within cellular processes. By delving into the depths of membrane channels, we aim to provide a comprehensive understanding of their pivotal role in governing cellular mechanics and biological harmony.
Unveiling Membrane Channels: Foundations and Functions
At the foundational level, membrane channels serve as gatekeepers that regulate the flow of ions and molecules across cellular membranes, dictating essential processes such as cell signaling, nutrient uptake, and cellular homeostasis. These dynamic conduits are intricately designed to facilitate the selective passage of specific molecules, thereby orchestrating a symphony of biochemical interactions vital for cellular survival and function. Understanding the diverse functions and structural adaptations of membrane channels is paramount to decoding the intricacies of cellular mechanics and signaling pathways.
The Architecture of Membrane Channels
The intricate architecture of membrane channels encompasses a myriad of structural motifs and protein configurations that govern their permeability and specificity. From ligand-gated channels to voltage-gated channels, each variant exhibits distinct structural features tailored to respond to different cellular cues and stimuli. Exploring the structural intricacies of membrane channels unveils a diverse landscape of protein arrangements, pore sizes, and gating mechanisms that underpin their functional versatility and regulatory precision within the cellular milieu.
Navigating the Functional Dynamics of Membrane Channels
Beyond their structural makeup, membrane channels intricately modulate cellular activities through precise spatial and temporal control of ion fluxes and molecule transport. The dynamic interplay between channel opening and closure finely tunes cellular responses to internal and external signals, orchestrating intricate processes such as action potentials, synaptic transmission, and muscle contractions. By elucidating the functional dynamics of membrane channels, we illuminate the regulatory mechanisms that underlie cellular communication, synaptic plasticity, and physiological equilibrium.
Regulatory Mechanisms of Membrane Channels
The regulatory mechanisms governing membrane channels exhibit a sophisticated interplay of ion gradients, channel kinetics, and modulatory factors that finely tune their activity in response to varying cellular conditions. From allosteric modulation to post-translational modifications, these regulatory cues exert exquisite control over channel gating, ion selectivity, and signal transduction pathways. Unraveling the diverse regulatory mechanisms of membrane channels provides a nuanced understanding of how cellular dynamics are intricately choreographed to maintain homeostasis and respond to environmental stimuli.
Synthesizing Insights: Illuminating Cellular Signaling Pathways
As we traverse the intricate world of membrane channels, a holistic synthesis of insights emerges, shedding light on the integrative role of these dynamic conduits in cellular signaling pathways and physiological responses. By deciphering the structural foundations, functional dynamics, and regulatory intricacies of membrane channels, we gain a profound appreciation for their indispensable contributions to cellular physiology, signaling cascades, and orchestrated responses to internal and external cues. This synthesis not only illuminates the critical role of membrane channels in cellular mechanics but also underscores their significance as fundamental players in the symphony of life.
Introduction to Membrane Channels
In the realm of cellular physiology, a fundamental aspect that reigns supreme is the intricate network of membrane channels that orchestrate crucial cellular functions. These microscopic gatekeepers play a pivotal role in regulating the passage of molecules in and out of cells, maintaining the delicate balance necessary for cellular homeostasis. Understanding the nuances of membrane channels is paramount in unraveling the mysteries of cellular physiology and comprehending the underlying mechanisms that drive biological processes.
Cell Membranes: The Gatekeepers of Cellular Function
Composition of Cell Membranes
Exploring the composition of cell membranes unveils a mosaic of lipids, proteins, and carbohydrates intricately arranged to form a structural barrier that safeguards cellular components. The lipid bilayer, a cornerstone of cell membranes, provides flexibility and dynamism, allowing cells to adapt to ever-changing external environments. Its hydrophobic core repels water-soluble substances while facilitating the passage of lipophilic molecules, ensuring selective permeability essential for cellular function.
Role of Liquid Bilayer in Cell Membrane Function
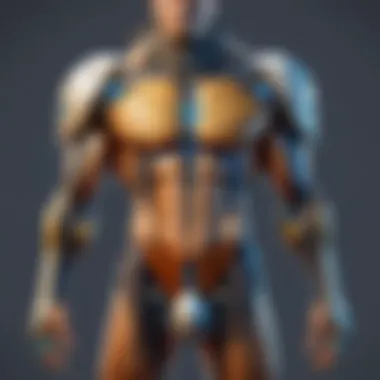
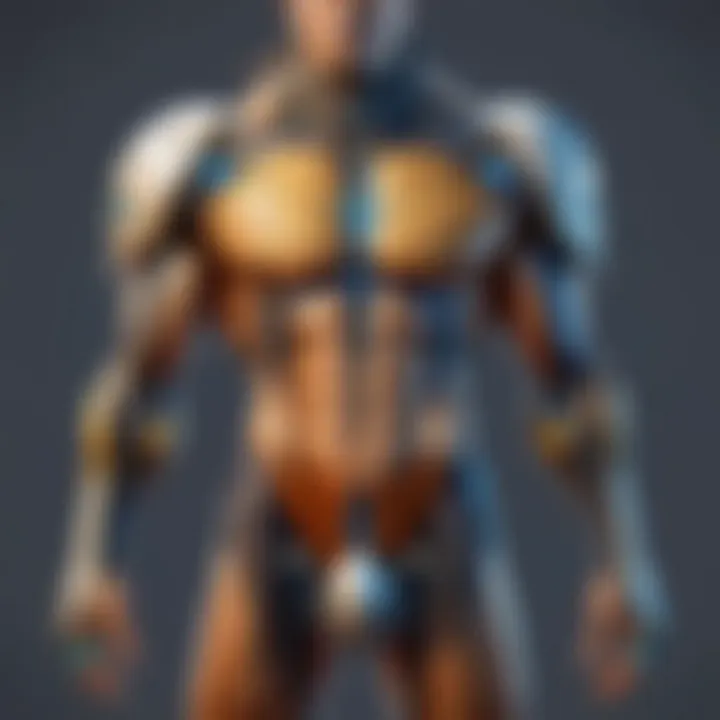
The lipid bilayer not only confers structural integrity to cell membranes but also serves as a platform for integral and peripheral membrane proteins that mediate a myriad of cellular processes. By segregating cellular compartments and acting as a boundary between the intra- and extracellular environments, the lipid bilayer regulates the flux of ions and molecules, orchestrating essential functions such as cell signaling and transport. Its fluidic nature enables membrane proteins to interact dynamically, fostering signal transduction and molecular transport critical for cellular homeostasis.
Key Concepts in Membrane Physiology
Selective Permeability
Selective permeability, a defining feature of membrane channels, dictates which molecules can traverse the cell membrane based on their size, charge, and solubility. This intricate mechanism allows cells to selectively uptake nutrients while excluding harmful substances, ensuring optimal cellular function. By utilizing transport proteins and ion channels, cells maintain osmotic balance and regulate intracellular signaling, showcasing the indispensable role of selective permeability in cellular physiology.
Transport Mechanisms Across Membranes
The transport mechanisms across membranes encompass a diverse array of protein-mediated processes that facilitate the movement of ions, molecules, and nutrients across the cell membrane. From passive diffusion to active transport, these mechanisms regulate nutrient uptake, waste expulsion, and signal transduction, enabling cells to respond to ever-changing physiological demands. Understanding the intricacies of transport mechanisms is paramount in dissecting the role of membrane channels in cellular physiology, shedding light on the dynamic interactions that govern cellular function.
Structure and Function of Membrane Channels
Structure and function of membrane channels play a vital role in the realm of cellular physiology. These intricate channels act as gatekeepers, regulating the flow of molecules in and out of cells, thereby maintaining cellular homeostasis. Understanding the structure and function of membrane channels is essential to grasp how cells communicate, transport essential nutrients, and remove waste products. With their selectivity and specificity, membrane channels ensure the precise movement of ions and molecules across cell membranes.
Proteins as Building Blocks of Membrane Channels
Proteins serve as the fundamental building blocks of membrane channels, dictating their structure and function. Within this context, two main categories of proteins contribute to the formation of membrane channels: integral membrane proteins and peripheral membrane proteins.
Integral Membrane Proteins
Integral membrane proteins are deeply embedded within the lipid bilayer of cell membranes. Their unique positioning allows them to span the entire membrane, serving as channels that facilitate the transport of specific molecules across the membrane. These proteins possess hydrophobic regions that anchor them securely within the lipid environment, ensuring their stability and functionality. One key characteristic of integral membrane proteins is their specificity in terms of the molecules they transport. This specificity makes them essential components of various cellular processes and signaling pathways.
Peripheral Membrane Proteins
In contrast, peripheral membrane proteins are not fully integrated into the lipid bilayer but are instead attached to the membrane's surface. These proteins play a crucial role in signaling and cellular recognition processes, facilitating interactions between the cell and its external environment. The key characteristic of peripheral membrane proteins lies in their ability to bind temporarily to the membrane, allowing for dynamic cellular responses. While integral membrane proteins are more stable and involved in long-term processes, peripheral membrane proteins exhibit rapid turnover and are essential for rapid cell signaling.
Channel Proteins: Gateway for Molecular Transport
Channel proteins act as the gateway for molecular transport across cell membranes, enabling the selective movement of ions and molecules in and out of cells. Ion channels and aquaporins are two prominent examples of channel proteins that play critical roles in cellular physiology.
Ion Channels
Ion channels are specialized protein pores that facilitate the passage of ions across cell membranes in a highly selective manner. By controlling the flow of ions, ion channels regulate crucial processes such as nerve signal transmission and muscle contraction. The key characteristic of ion channels is their ability to open and close in response to specific signals or changes in membrane potential, allowing for precise control over ion movement. While ion channels are vital for maintaining cellular function, dysfunction in these channels can lead to various neurological and cardiovascular disorders.
Aquaporins
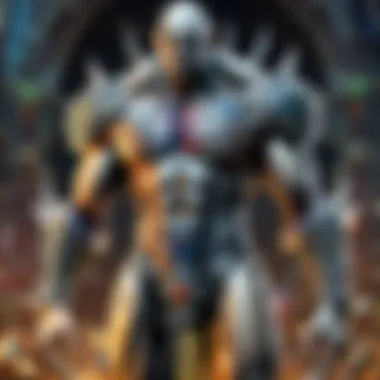
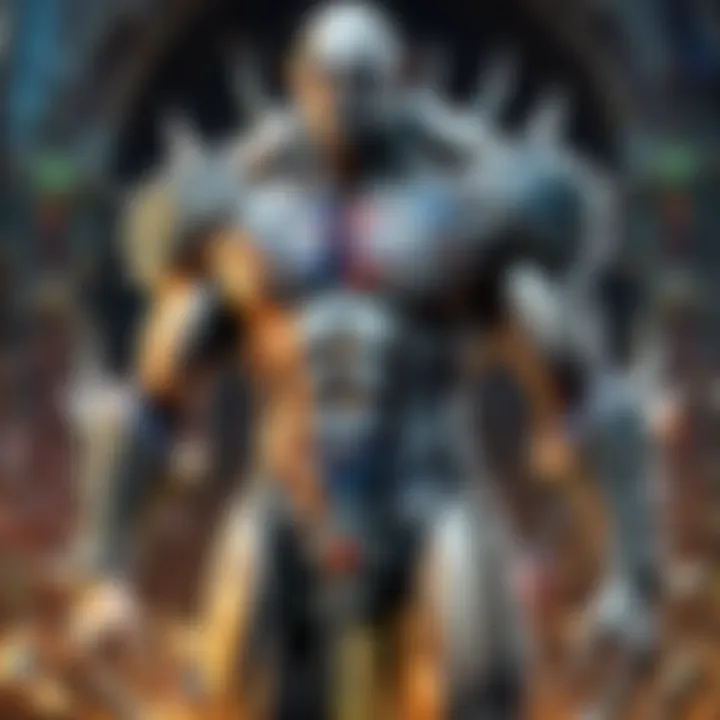
Aquaporins are a class of channel proteins that specifically facilitate the transport of water molecules across cell membranes. These proteins play a pivotal role in maintaining water balance within cells and tissues, ensuring proper hydration and osmoregulation. The key characteristic of aquaporins is their high permeability to water while excluding other molecules, making them essential for rapid water transport. Although primarily associated with water transport, aquaporins also contribute to the movement of small solutes, highlighting their versatility in cellular processes.
Regulation and Significance of Membrane Channels
In this section, we delve into the pivotal topic of Regulation and Significance of Membrane Channels within the context of cellular physiology. Membrane channels play a crucial role in orchestrating various cellular functions and maintaining homeostasis. Understanding how these channels are regulated is essential for grasping their significance in cellular processes. By exploring the mechanisms that govern the activity of membrane channels, we gain insight into how cells communicate internally and with their environment.
Dynamic Regulation of Ion Channels
Voltage-Gated Ion Channels
Voltage-gated ion channels are a specific type of membrane channel that respond to changes in membrane potential. This unique characteristic allows them to regulate the flow of ions across the cell membrane in a voltage-dependent manner. Their contribution to cellular function lies in their ability to generate and propagate action potentials, important for nerve conduction and muscle contraction. Voltage-gated ion channels are favored in this article due to their critical role in physiological processes and their potential as therapeutic targets for various diseases.
With their distinctive feature of opening and closing in response to alterations in membrane voltage, voltage-gated ion channels precisely control the movement of ions such as sodium, potassium, and calcium. This property enables them to modulate cellular excitability and regulate important biological functions. While their precise gating mechanism ensures rapid and precise ion flux, the complexity of their regulation poses challenges in therapeutic targeting, necessitating detailed study.
Ligand-Gated Ion Channels
Ligand-gated ion channels are another key player in cellular signaling, responding to specific chemical messengers or ligands to mediate ion passage across the membrane. Their activation by ligands such as neurotransmitters or hormones enables rapid cellular responses to external stimuli. The presence of ligand-gated ion channels in this article is attributed to their widespread involvement in synaptic transmission, sensory perception, and muscle contraction.
The defining feature of ligand-gated ion channels is their ability to alter ion permeability upon binding of specific molecules, resulting in changes in membrane potential and cellular excitability. This swift response mechanism allows for rapid signal transduction and precise control over physiological functions. While their efficient communication role is advantageous for swift cellular responses, the susceptibility to desensitization and functional modulation presents challenges in sustaining long-term signaling processes.
Physiological Implications of Membrane Channels
Nerve Signal Transmission
Nerve signal transmission relies on the coordinated action of ion channels to propagate electrical impulses along neurons. This communication process is essential for brain function, sensory perception, and motor control. By understanding the mechanisms underlying nerve signal transmission, we gain insights into neural connectivity and information processing within the nervous system.
The key characteristic of nerve signal transmission is its reliance on ion channels to facilitate the rapid transfer of signals between neurons. This process involves sequential opening and closing of ion channels along the neuronal membrane, allowing for the generation of action potentials that travel along nerve fibers. While this efficient mode of communication enables swift responses to stimuli, disruptions in ion channel function can lead to neurological disorders and impaired nerve transmission.
Muscle Contraction
Muscle contraction, a fundamental physiological process, is driven by the intricate interplay of ion channels in skeletal and cardiac muscle cells. The contractile machinery within muscle fibers relies on the precise regulation of calcium ions through specialized membrane channels. Exploring the role of ion channels in muscle contraction unravels the mechanisms that underlie movement, strength, and muscle coordination in vertebrates.
The paramount characteristic of muscle contraction is its dependence on calcium ion influx through membrane channels to trigger the molecular events leading to muscle fiber shortening. This calcium-induced activation process is crucial for force production and muscle movement. While the tight regulation of calcium ion concentration ensures precise contractile activity, abnormalities in ion channel function can result in muscle weakness, spasms, or even life-threatening conditions.
This detailed exploration of ion channel dynamics in nerve signal transmission and muscle contraction sheds light on their indispensable roles in cellular physiology and opens avenues for understanding and treating associated disorders and diseases.
Diseases and Disorders Linked to Membrane Channel Dysfunction
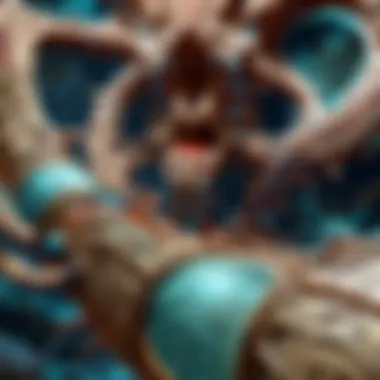
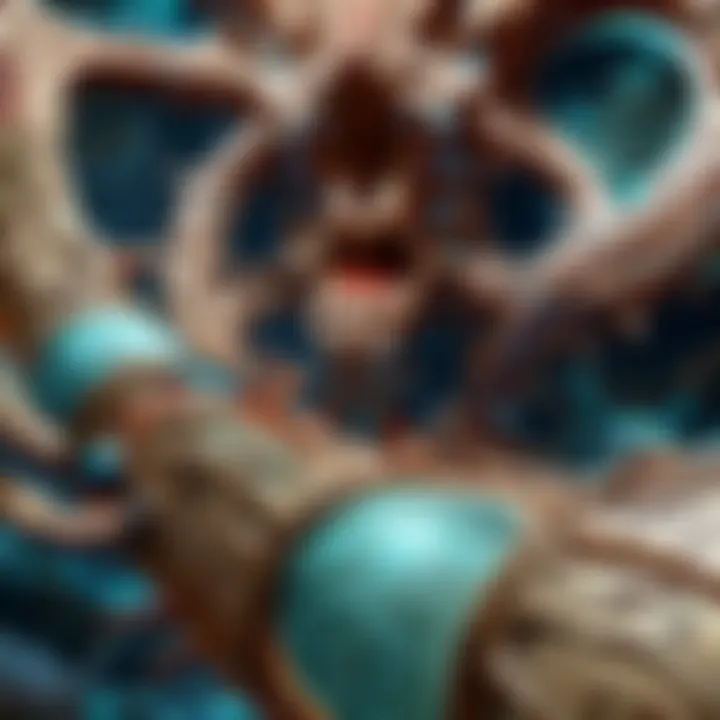
Diving deep into the realm of membrane channels reveals a crucial aspect of cellular physiology - the link between membrane channel dysfunction and various diseases and disorders. Understanding this connection sheds light on the intricate mechanisms underlying cellular processes and highlights the significant role of membrane channels. By examining how malfunctions in these channels can lead to disruptions in cellular function, we can appreciate the impact of membrane channels on overall health and well-being.
Channelopathies: Unraveling Disease Connections
Channelopathies are a group of disorders characterized by dysfunction in ion channels, leading to various health issues. Among these conditions, Cystic Fibrosis stands out as a prominent example of a disease linked to membrane channel dysfunction. This genetic disorder primarily affects the lungs and digestive system, resulting in mucus buildup and impaired organ function. Understanding the molecular basis of Cystic Fibrosis provides valuable insights into membrane channel physiology and the consequences of channel malfunction in disease development.
Cystic FibrosIs
Cystic FibrosIs, despite being a challenging term to grasp, encapsulates the essence of a debilitating genetic disorder. The distinctive feature of Cystic FibrosIs lies in its impact on the respiratory and digestive systems, where the abnormal secretion of thick, sticky mucus obstructs airways and disrupts digestion. Exploring Cystic FibrosIs within the context of membrane channel dysfunction sheds light on the intricate interplay between ion transport and cellular pathology, making it a pertinent subject for in-depth analysis within this article.
Long QT Syndrome
On the other hand, Long QT Syndrome presents a different facet of membrane channel dysfunction. This rare heart condition affects the heart's electrical activity, predisposing individuals to dangerous arrhythmias and sudden cardiac arrest. Unveiling the intricacies of Long QT Syndrome in the context of membrane channels offers a glimpse into how channel abnormalities can have far-reaching consequences on physiological processes, emphasizing the significance of channelopathies in understanding disease mechanisms.
Ion Channel Blockers
In treating disorders associated with membrane channel dysfunction, Ion Channel Blockers emerge as key therapeutic agents. These medications target specific ion channels to modulate their activity, effectively restoring normal cellular function. The unique characteristic of Ion Channel Blockers lies in their precision targeting of dysfunctional channels, highlighting their potential in managing channelopathies and related conditions. By delving into the mechanisms of action of Ion Channel Blockers, we can garner insights into the intricate interplay between drugs and membrane channels for therapeutic purposes.
Channelopathy Treatments
Furthermore, Channelopathy Treatments encompass a range of therapeutic approaches aimed at mitigating the effects of channel dysfunction on cellular physiology. These treatments may include gene therapies, ion channel modulators, or other interventions targeting the underlying molecular defects. Exploring the nuances of Channelopathy Treatments within the context of membrane channel dysfunction offers a holistic view of how medical interventions can address the root causes of channelopathies, paving the way for more targeted and effective treatment strategies within the realm of cellular physiology.
Future Perspectives and Research Directions
Emerging Trends in Membrane Channel Research
CRISPR-Cas9 Techniques in Studying Membrane Channels
Undoubtedly, one of the most intriguing aspects within membrane channel research is the integration of CRISPR-Cas9 techniques. These cutting-edge tools have revolutionized the study of membrane channels by allowing for precise genetic modifications. The specificity and efficiency of CRISPR-Cas9 in manipulating membrane channel genes open up new avenues for groundbreaking discoveries. Researchers can now target and modify specific channels with unparalleled precision, offering insights into their functions and regulatory mechanisms. However, the implementation of CRISPR-Cas9 techniques introduces challenges such as off-target effects and ethical considerations that necessitate careful consideration.
Nanotechnology Applications in Membrane Channel Studies
Nanotechnology applications play a pivotal role in enhancing our understanding of membrane channels. Through nanoscale technologies, researchers can visualize, manipulate, and measure membrane channels with unprecedented precision. The unique characteristic of nanotechnology lies in its ability to probe cellular processes at a molecular level, shedding light on intricate channel dynamics that were once elusive. Leveraging nanotechnology in membrane channel studies offers new perspectives on channel behavior and interactions within cellular environments. Despite its advantages, the application of nanotechnology also presents challenges related to standardization, reproducibility, and potential impacts on biological systems.
Challenges and Opportunities in Membrane Channel Exploration
Understanding Channel Diversity
The exploration of channel diversity emerges as a key aspect in unraveling the complexities of membrane channels. Understanding the diverse range of channels expressed in cells is essential for comprehending their roles in physiological processes. By dissecting channel diversity, researchers can uncover hidden mechanisms underlying cellular functions and pathologies. The key characteristic of this exploration lies in its potential to unveil novel channel subtypes with distinct properties and functions. However, navigating the vast landscape of channel diversity presents challenges in categorization, functional categorization, and integration of emerging data sources.
Exploring Novel Therapeutic Targets
Exploring novel therapeutic targets within membrane channels offers promise in advancing personalized medicine and drug development. By targeting specific channels implicated in diseases, researchers can design tailored interventions to modulate channel activity and restore cellular homeostasis. The key characteristic of this endeavor is the quest for precision medicine strategies that cater to individual variations in channel function. While the pursuit of novel therapeutic targets holds immense potential, it is accompanied by challenges related to target validation, drug specificity, and potential side effects that necessitate rigorous testing and validation processes.
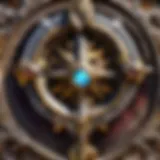
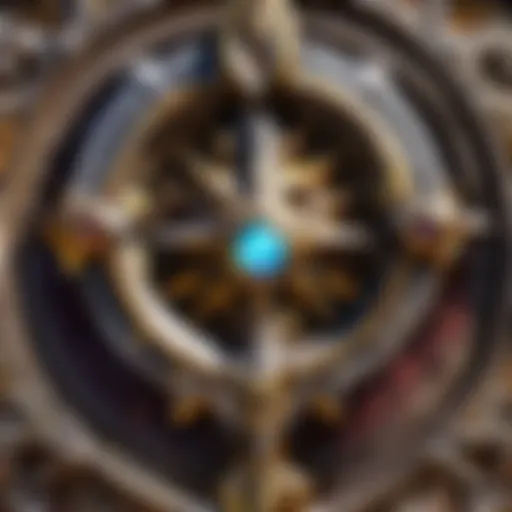